From the fluttering wings of moths, the leaping antelopes, to the undulating snakes and nematodes... various creatures produce movement through a combination of neural and mechanical controls.
Animals integrate and process sensory information through neural circuits, generating movement commands and executing precise physical actions through complex networks of signals.
Animals rely not only on neurons (such as brain cells and peripheral nerves) to control movement but also utilize the physical properties of their bodies (such as the elasticity of muscles) to help them spontaneously respond to the environment before the neurons have a chance to react.
Therefore, from the perspective of how biological systems generate movement, it can be considered that "embodied intelligence" is mainly composed of two parts—"computational intelligence" and "mechanical intelligence".New Understanding: "Mechanical Intelligence" is an Important Part of "Embodied Intelligence"
"Embodied intelligence" is a concept that has been very popular in the field of robotics in recent years. As a doctoral student in robotics who studies bionic robots, I am also a researcher in the interdisciplinary field of biomechanics, biophysics, robotics physics, and more.
Advertisement
Wang Tianyu from the Georgia Institute of Technology demonstrated in the latest research the unique understanding and interpretation of mechanical intelligence and embodied intelligence in biological motion by his team.
Recently, the relevant paper was published in Science Robotics (IF=34.0) with the title "Mechanical intelligence simplifies control in terrestrial limbless locomotion" [1].
Wang Tianyu and Christopher Pierce, a postdoctoral fellow at the Georgia Institute of Technology, are the co-first authors, and Professor Daniel I. Goldman from the Georgia Institute of Technology is the corresponding author.This study has been affirmed by peers and experts from fields such as biology, physics, and robotics, who believe that this research has the potential to bring the study of limbless creature movement, as well as the design and control of limbless robots in complex terrains, to a whole new level. It opens up more new possibilities for a deeper understanding of the limbless movement of generalized transverse systems and how to improve the performance of robots by utilizing the principles learned from biology.
Based on a unique understanding of "embodied intelligence," Wang Tianyu has extended the concept of "mechanical intelligence" to the study of the movement of bionic robots.
Taking the limbless robot (also known as the snake robot) in this study as an example, by bending each part of the body to the left and right, this kind of movement is called "lateral undulation movement." If the robot collides with an obstacle, they must turn or bypass the obstacle by adjusting their body posture.
To complete this task, one can design an algorithm or control system, or a sophisticated physical system.
For example, by installing force sensors on the robot's head or body, an algorithm can be designed to make the robot move away from or bypass obstacles when the head or body senses a sufficiently large force. This, in turn, endows the robot with a certain degree of "computational intelligence."Alternatively, by designing the way robots drive their bodies and selecting the materials for making the robot's body, the robot can spontaneously generate body deformations when colliding with the environment to deal with obstacles without "thinking". In this way, the robot has "mechanical intelligence".
The secret of "mechanical intelligence" lies in the driving mode.
Apod locomotion is a common mode of movement in the natural world, occurring in various different and usually highly complex heterogeneous environments, and spanning length scales and neural system complexity.
From snakes that are several meters long with more than a million neurons to the microscopic millimeter-level Caenorhabditis elegans with only 302 neurons.When these biological systems enter unfamiliar and complex environments (such as snakes in the forest, and nematodes in rotting fruit), they do not need any time to observe or learn about their surroundings, and can spontaneously move freely within the environment.
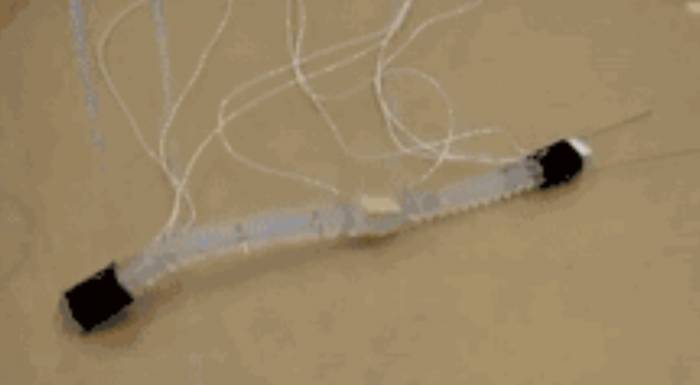
In the field of biomechanics, scientists have made significant progress in understanding the "computational intelligence" of such movement control, including the structure, function, and dynamics of neural circuits.
However, having computational intelligence alone is not enough. The "mechanical intelligence" hidden within the bodies of these biological systems is also an indispensable part of their ability to complete such incredible movement processes.
Currently, there is still a lack of research on the mechanical intelligence of limbless biological systems. One of the main reasons is that it is difficult for scientists to separate the body's functions from the nervous system and study the mechanisms of the biological body in motion under completely blocked neural activity.
This leads to the significance of developing robotic systems for the study of biological system movement - by constructing a "robotic physics" model, that is, developing a specific type of robotic system for studying the mechanisms of biological movement.Scientists can perform experiments in robotic systems that are difficult to accomplish in biological organisms. For example, it is extremely easy to make a snake-like robot turn 90 degrees, but controlling a snake to turn 90 degrees is nearly impossible.
When comparing the most cutting-edge limbless robotic systems today with the common limbless biological systems found in nature, a very significant difference is evident in the way the body is driven: in almost all limbless robots, the rotation of each joint is achieved by a rotating motor.
In limbless biological systems, the bending of the body is achieved by driving the contraction of muscles on both sides of each joint. Wang Tianyu realized that the secret of mechanical intelligence might be hidden in the driving mode.
In light of this, he and his team designed and manufactured a new type of limbless robot called MILLR (Mechanically Intelligent LimbLess Robot).
Each joint of MILLR is bent by two independently controlled ropes, which can pull each joint to the left and right sides.When a rope is taut, it can actively pull the joint towards one side; whereas when a rope is relaxed, the joint on that side is no longer constrained and can passively adapt to the forces exerted on it by the environment.
By planning and coordinating the tension-relaxation movements of each rope, programmable, quantifiable, dynamic, and anisotropic body compliance can be achieved on MILLR.
When a robot collides with an obstacle, depending on the state of body compliance, the robot can selectively maintain its body shape or passively bend under the influence of the force exerted by the obstacle.
Thanks to such body compliance, many collisions that would have caused the robot to stop moving or get stuck now naturally cause the robot to deform and conform to the obstacle, allowing the robot to continue propelling its body forward.
When testing MILLR in a multi-obstacle environment model set up in the laboratory, Wang Tianyu found that too low body compliance would cause MILLR to get stuck by the obstacle, while too high compliance would cause the body to not maintain the required undulating posture, thus failing to provide sufficient power to move forward.Only when MILLR is endowed with moderate body flexibility can it spontaneously move forward in an obstacle environment under open-loop control, without any perception of the environment and active body posture adjustment, which is also a manifestation of mechanical intelligence in MILLR.
At the same time, through robot experiments and comparisons with the nematode Caenorhabditis elegans in similar environments, it was found that when MILLR has moderate body flexibility, both the open-loop speed performance and body kinematics are very similar to that of the nematode.
This also verifies a biological hypothesis: relying solely on mechanical intelligence is enough to enable limbless systems to deal with obstacles encountered in motion.
Finally, by installing head collision sensors for MILLR, the function of the "retreating" behavior of nematodes during motion was experimentally explored. At the same time, the research team found that appropriate computational intelligence can compensate for the shortcomings of mechanical intelligence, or complement mechanical intelligence, thereby enhancing motion performance.
So far, the robot system MILLR has become an example of embodied intelligence, demonstrating the most powerful motion capabilities when working under the collaborative effect of mechanical intelligence and computational intelligence.In summary, this study highlights the role of mechanical intelligence in shaping the movement of limbless systems, providing in-depth insights into the interplay between neural and mechanical control in nematodes and other limbless animals.
At the same time, through the robotic MILLR, the team has validated the significant role of mechanical intelligence in the movement of limbless robots, with the hope of further advancing the application of limbless robots in fields such as search and rescue, industrial inspection, agriculture, and planetary exploration.
Starting as a "side project," it has formed a closed loop of "robotics-biology."
It is also reported that Wang Tianyu studied electronic and computer engineering at the University of Michigan-Shanghai Jiao Tong University Joint Institute, then obtained a master's degree in mechanical engineering from Carnegie Mellon University in the United States, and is currently pursuing a Ph.D. in robotics at the Georgia Institute of Technology, focusing on the movement of limbless and multi-legged robots inspired by biology in complex environments.He mentioned that the research initially stemmed from a topic in his master's research, which was how to design control algorithms to make a snake-like robot have body flexibility to cope with complex environmental terrains. At that time, he achieved this goal with thousands of lines of code.
However, when he saw that living organisms could seemingly effortlessly achieve this goal, he began to think: do they not "think" so much when they move? So what makes it so easy for them to achieve what computers can only do with thousands of lines of code?
After he joined the Georgia Institute of Technology to pursue a Ph.D. in robotics, he found that there had been related research within the research group, namely how snakes use muscle drive and the passivity of the body to overcome obstacles. So, he came up with the idea of further making a robot to simulate the way snakes drive their bodies with muscles.
After communicating with his mentor, his mentor thought this direction was worth exploring, but because there was no special fund for this robot project at the time, he agreed to try it as a "side job".
With the continuous deepening of research and the joining of some like-minded partners, Wang Tianyu and his team found that some behaviors and body forms of the snake-like robot they designed were very similar to the nematodes they observed.
(Note: The original text seems to be cut off or incomplete, so the translation ends accordingly.)Thus, the project further evolved. It is not only about bio-inspired robot design, but also about using robots as experimental tools to better understand the movement of living organisms, thereby forming a "robotics-biology" feedback loop.
Despite the unparalleled mobility of wheeled and legged robots, there are still some special environments in practical application scenarios where these traditional mobile robots struggle to be effective. For example, in the debris of collapsed buildings, in complex pipe systems, in granular media such as soil, sand, and snow, or in extreme environments like ice crevasses.
However, in such environments, limbless robots can utilize their elongated bodies for movement to accomplish a series of tasks such as search and rescue, industrial inspection, agricultural management, and even geological exploration on other planets.
Understanding the role of mechanical intelligence in limbless movement not only helps them to gain a deeper understanding of the movement of living organisms but also aids in designing and manufacturing limbless robots that can better adapt to complex environments without the need for complex sensing and control algorithms.In the future, in the field of robotics, he hopes to further expand the application scope of mechanical intelligence principles, including the realization of manufacturing 3D robots that can perform more gaits, such as lateral bending, accordion style, and so on.
Additionally, they are also developing waterproof versions to explore the research field of limbless underwater robot motion.
In terms of biology, the team hopes to further study the principles of muscle-tendon drive in biological bodies, deepening the understanding and quantification of mechanical intelligence in biological systems. Moreover, the mechanical intelligence principles discovered and verified in this study are expected to extend to more limbless biological systems, such as snakes and eels.
POST A COMMENT