Recently, a research team led by Associate Professor Wang Chong from the City University of Hong Kong has developed an integrated microwave photonic processor based on a thin-film lithium niobate platform, marking the first time that an ultrafast electro-optic conversion module and a low-loss, multifunctional signal processing module have been combined on the same chip.
This lithium niobate microwave photonic chip, which efficiently performs analog signal processing and computational tasks, can achieve an ultra-wide processing bandwidth of 67GHz and high-precision computation of 96.6%. In terms of speed, it is 1000 times faster than traditional electronic processors, and it consumes less energy.
Advertisement
The technology has shown potential for application in various fields, including 5G/6G wireless communication systems, high-resolution radar systems, artificial intelligence, computer vision, and image/video processing.
The reviewers of the study commented, "This research represents a valuable technological advancement in this field. The results strongly demonstrate the significant performance advantages of the lithium niobate photonic processor proposed by the authors."
Recently, the related paper, titled "Integrated lithium niobate microwave photonic processing engine," was published in Nature[1]. Feng Hanke, a doctoral student at the City University of Hong Kong, and Ge Tong, an undergraduate student, are the co-first authors, and Associate Professor Wang Chong serves as the corresponding author.1000 times faster than traditional electronic processors
Currently, with the rapid expansion of fields such as wireless communication networks, autonomous driving, the Internet of Things, big data, and cloud services, combined with the surge of artificial intelligence, a vast amount of data information has put forward higher demands for signal processing systems.
So, how can we achieve processors with larger bandwidth, higher processing speed, and lower power consumption? To break through the bottleneck of speed and bandwidth of traditional electronic processors, photonic processors have emerged.
As the name suggests, photonic processing chips use light to perform signal processing and computational tasks. Compared with traditional electronic chips, light has faster speed, higher computational efficiency and throughput, and less energy loss during light transmission, which does not generate too much heat, thereby reducing energy consumption.The study was able to achieve such outstanding results, thanks to the unique advantages of lithium niobate in the field of photonics. The importance of lithium niobate to photonics is comparable to that of silicon in microelectronics, which is why it is also known as the "silicon of photonics."
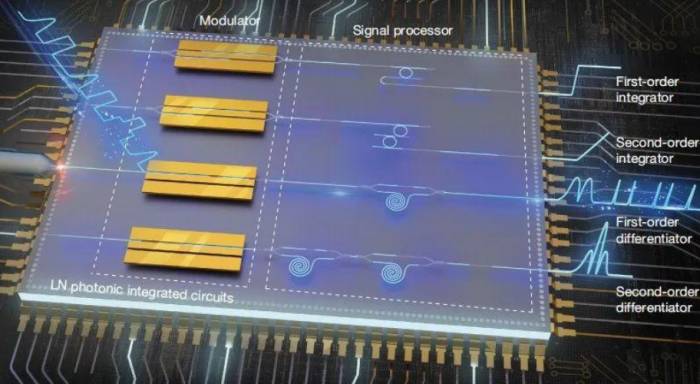
Lithium niobate's unique electro-optic properties, ultra-low optical loss, and large-scale, low-cost manufacturing processes make it stand out in building ultra-fast photonic processing chips, becoming the ideal material for achieving high-speed and low-consumption chips.
At the beginning of the study, due to the newly established laboratory of Wang Cheng, how to quickly solve the lithium niobate wafer process problem became their biggest challenge. The lithium niobate process is a perennial issue, and a good process is the foundation for research and the necessary premise for preparing good devices.
"In the initial stage, I spent every day in the cleanroom exploring the process, and then adjusted and improved the process flow by combining the morphological electron micrograph of the etched lithium niobate waveguide. As a research 'newcomer,' it is inevitable to encounter difficulties," said Feng Hanke.
This is a time-consuming, tedious but essential process. They proposed to achieve the ultra-high-speed time signal integration function by integrating the intensity modulator and micro-ring resonators with high quality factors on the same chip. At that time, the members of the research group would discuss every day how to optimize the entire process flow, and then get more and more ideal test results through adjustments time and again.Researchers have further realized the function of second-order integration through cascaded microring resonators. "When we observed the results of ultra-fast signal processing from the experiment, we realized that this technology has a very broad application potential, so we began to explore possible application scenarios," said Ge Tong.
Based on this integrator, the research team demonstrated the application of microwave photonics—solving ordinary differential equations at ultra-high speed. Researchers adjusted the quality factor of the microring resonator, which allowed for solving equations under different parameters, with a calculation accuracy of 98.1%. Compared with traditional electronic processors, this system has obvious advantages, significantly improving processing speed.
In addition, the team also realized an ultra-high-speed differentiator based on frequency chirping. They used a signal generator to directly input an ultra-short sinc pulse with a duration of 9.6 picoseconds into the photonic chip, effectively and accurately obtaining the differentiation results. The realization of this result also demonstrates the ultra-high-speed signal processing capability of the lithium niobate microwave photonic chip.
Wang Chong said, "Inspired by a paper in Nature Photonics, I had in-depth discussions with everyone and decided to use the developed optical processing chip for image edge detection. We established cooperation with Professor Yuan Yixuan from the Chinese University of Hong Kong and Dr. Guo Xiaoqing from the University of Oxford in the UK, and quickly started research in related fields."
In this study, researchers input the "CityU" icon with 250x250 pixels into the chip in the form of a data stream, and obtained differentiation information at a processing speed of 2560 billion pixels per second.Ultimately, they were pleasantly surprised to find that compared to existing technologies, the microwave photonic chip not only maintained a high accuracy of 96.6%, but also completed the same task with extremely low power consumption and at a thousandth of the original time (about three orders of magnitude faster).
On the other hand, they also explored for a long time on how to characterize the high performance of the chip. After the first round of review, the reviewers pointed out some shortcomings in the paper. Researchers needed to develop new chips and formulate new experimental schemes to elaborate and highlight the advantages of lithium niobate microwave photonic chips. However, at that time, the shortage of testing equipment greatly limited the feasibility of the experimental scheme.
After several months of discussion, communication, and debugging, with the help of Professor Huang Chaoran from the Chinese University of Hong Kong and doctoral student Wang Benshan, the team rebuilt a high-speed testing experimental platform, fully verifying the performance advantages of lithium niobate chips.
From a scientific research "newcomer" to being responsible for the entire group's chip processing, Feng Hanke recalled: "I remember the first time I made a lithium niobate chip in my life, and even light couldn't pass through it. Later, I continuously updated the quality factor (Q value) of the micro-ring resonator in the laboratory, and every breakthrough brought a sense of achievement."
Although this research process was full of hardships and challenges, when the ideal experimental results finally appeared on the oscilloscope, the entire team felt that all the efforts were worthwhile.The Prospects of Large-Scale Commercialization in the Field of Optical Communication
Driven by the wave of big data and AI, the global demand for computing power will continue to grow rapidly. The upgrade of optical module technology is not only about doubling the speed, but also about addressing the high power consumption and high costs brought about by the increase in speed.
Lithium niobate thin-film optical modulators have an extremely high cost-performance ratio, meeting the speed requirements while also having low power consumption. Wang Cheng pointed out that the commercialization process of modulator technology based on thin films has been going on domestically and internationally for some time, and is expected to achieve large-scale commercial application in the field of optical communication within the next one or two years.
The research group believes that the greater advantage of the lithium niobate thin-film platform in the future lies in its ability to provide high-speed electro-optic control, low loss transmission, and scalability when applied to larger scale on-chip systems. "These are not available in other mainstream platforms at present, and are also the main direction of our efforts," said Feng Hanke.When a chip provides more than just a single device, it has more room for development, and potential application scenarios include wireless communication, the Internet of Things, millimeter wave radar, and other fields.
Taking the system in this study as an example, the 6G network will need to operate in the millimeter wave band (30-300GHz) at frequencies much higher than the current 5G network (~5GHz), and it will require more base stations to support signal transmission, reception, and scheduling.
Since the coverage of each base station is much smaller, the devices that support such networks need to operate at higher frequencies, and therefore should also meet the needs for more integration, lower power consumption, and lower cost.
Signal processors based on traditional electronic chips find it difficult to operate efficiently at these frequency bands, and there are significant challenges in terms of power consumption, noise, and bandwidth. Wang Cheng said: "Our lithium niobate microwave photonic processing chip transfers these difficulties to the optical frequency band, which has an almost unlimited available bandwidth for processing, thus providing a solution with greatly improved performance."The development and validation of the industrial applications of lithium niobate thin film technology will continue.
Wang Cheng obtained a bachelor's degree in microelectronics from Tsinghua University, and master's and doctoral degrees in electrical engineering from Harvard University, studying under Professor Marko Lončar, and continued to engage in postdoctoral research at Harvard University.
In 2018, Wang Cheng collaborated with a team from Harvard University and Nokia Bell Labs to develop the world's first integrated electro-optic modulator compatible with voltage and complementary metal-oxide-semiconductor (CMOS) chips on a lithium niobate platform [2], thus igniting a "boom" in the research of lithium niobate thin films.
In the same year, Wang Cheng established an independent research group at City University of Hong Kong, continuing to delve into the field of integrated lithium niobate photonics and exploring high-performance wafer-level processing techniques for lithium niobate.
Previously, the team's research had ventured into the field of microwave photonics. For example, the research on high linearity modulators and millimeter wave-photonic modulators, respectively, addressed significant bottlenecks in the application of the lithium niobate thin film platform to microwave photonics and millimeter wave photonics, improving the link's linearity and expanding the system's response [3-4] (DeepTech previous report: Providing new solutions for microwave and millimeter wave photonics bottlenecks, the City University of Hong Kong team greatly enhances the linearity and operating bandwidth of lithium niobate modulators).Due to "the invention of a unique lithium niobate thin-film platform and a supporting micro-nano processing system, achieving high-performance, compact, low-cost, and low-power optical interconnection solutions," Wang Chong became one of the Chinese region's selected individuals for the 2021 MIT Technology Review "35 Innovators Under 35" (DeepTech previous report: Wang Chong: Breaking through traditional bottlenecks, developing high-performance integrated lithium niobate optoelectronic chips, achieving high-performance and low-cost optical interconnection solutions).
Based on the new research results, they plan to continue to advance in the further scaling of the lithium niobate process and the further optimization of the packaging process, in order to reduce the insertion loss of the entire device, improve work stability, and further achieve larger-scale on-chip signal processing, so as to truly test the application effect of the entire chip in the field system.
In the next phase, they plan to further enhance the integration and functionality of the lithium niobate on-chip optical system. This includes not only integrating with other functional devices in the research group (such as optical frequency combs) on the same chip but also heterogeneous integration with other optical materials to achieve the integration and synergistic effect of more devices including lasers and detectors.
In terms of industrial cooperation, Wang Chong and his team are currently actively exploring cooperation with optical communication equipment manufacturers and optical device suppliers to jointly develop and verify the application of thin-film lithium niobate technology in related industries. He said: "We also welcome more interested industrial partners to contact us."
POST A COMMENT