High product selectivity electro-oxidation of primary alcohols to carboxylic acids is an important industrial catalytic reaction, commonly used in the production of bulk and fine chemicals.
However, there are three major challenges in understanding the oxidation of alcohols by nickel-based catalysts:
1. The rate-determining step of the electrocatalytic oxidation reaction is whether it is the oxidation of alcohols or the formation of Ni3+OOH.
2. The electron transfer mechanism follows the redox process of Ni2+(OH)2/Ni3+OOH, or is it a non-redox process of direct electron transfer of Ni3+?
3. How to understand the product selectivity of alcohol electro-oxidation?For many years, Professor Yan Shicheng from Nanjing University and his research group have been committed to revealing the catalytic mechanism of organic electro-oxidation by nickel-based materials, taking the oxidation of various primary alcohols (methanol, ethanol, propanol, butanol, benzyl alcohol) to carboxylic acids as an example, to reveal the rate-determining steps, electron transfer mechanisms, and product selectivity mechanisms of organic electrocatalytic oxidation reactions.
Advertisement
To clarify the rate-determining step of the organic electrocatalytic oxidation reaction, they compared the cyclic voltammetry curves of water oxidation and methanol oxidation, in-situ electrochemical Raman spectra, and in-situ electrochemical UV-visible absorption spectra, confirming that the rate-determining step of the methanol oxidation reaction is the generation of Ni3+, and Ni3+ is the catalytically active species.
To clarify the electron transfer mechanism of organic electrocatalytic oxidation, they combined experiments with theory to clarify the direct electron transfer mechanism of the electrochemical interface Ni3+ active center.
In terms of experiments, they used dinitrophenyl hydrazine as a highly sensitive Ni2+ complexing agent (monitoring concentration limit nM, time scale ns), clarifying whether the electron transfer of the Ni3+ catalytic center goes through the Ni3+/Ni2+ valence change process.
The research found that when the voltage is rapidly switched within the voltage range of Ni3+ generation, the electrode continuously oxidizes methanol and transfers a large number of electrons, and at this time, Ni2+ cannot be detected.However, during the cyclic voltammetric scan within the Ni2+/Ni3+ oxidation-reduction voltage range, the detection of Ni2+ can be observed, which implies that during the methanol oxidation process, the electron transfer from Ni3+ does not go through an intermediate valence state of Ni2+.
The sudden drop in electrochemical impedance spectroscopy after the generation of Ni3+ and the activation energy of 0 for the oxidation of methanol by Ni3+ further prove that the electron transfer of Ni3+ is a semiconductor-like behavior.
In terms of theory, the premise of understanding the electron transfer mechanism at the electrochemical interface is to clarify the theory of electron transfer in electrochemical reactions.
Canadian chemist Rudolph A. Marcus, based on the isoenergy electron macroscopic tunneling effect at the electrode/electrolyte interface and the Franck-Condon principle, which recognizes that the time scale of electron transfer is much faster than the change in molecular configuration, proposed the Marcus charge transfer theory between 1955 and 1965 (later awarded the Nobel Prize in Chemistry in 1992).
The aforementioned theory clearly states that the electrolyte in electrochemical reactions can be regarded as a continuous dielectric environment, and the fluctuation of the reaction molecule's nuclear coordinates originates from the interaction between the reaction molecule and the dielectric environment, as well as with the active center of the electrode.The nuclear coordinate fluctuation is the fundamental premise for chemical reactions to satisfy the principles of energy conservation and the Frank-Condon principle, as well as establishing the basis for isoenergetic electron tunneling.
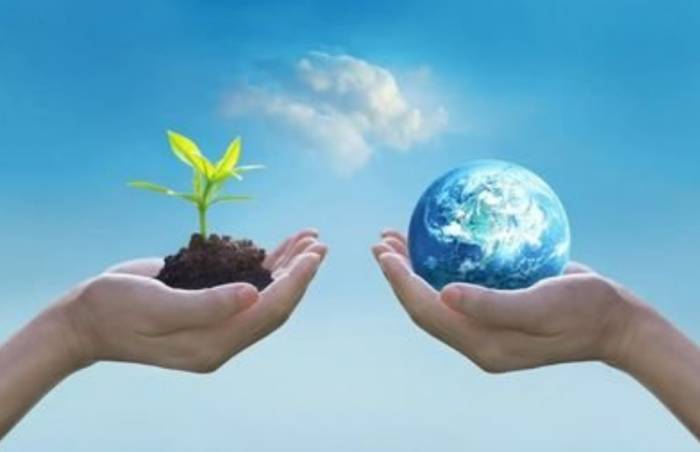
Under the action of external voltage, the electrode catalytic center is gradually polarized to the isoenergetic electron transfer state. At the same time, the reaction molecules interact with the electrolyte environment and the electrode catalytic center, causing their nuclear coordinate fluctuations to reach the isoenergetic electron transfer state, thus the electrochemical reaction adheres to the principles of energy conservation and the Frank-Condon principle.
According to Marcus's electron transfer theory, when the electrode polarizes Ni2+ to the isoenergetic electron transfer state of Ni3+, methanol molecules will also adjust their geometric configuration through solvation effects and interactions with the active center to reach the isoenergetic electron transfer state, thereby overcoming the Frank-Condon barrier (activation energy) to facilitate isoenergetic macroscopic electron transfer.
It can be seen that from the perspective of Marcus's electron transfer theory, in the process of electron transfer in the oxidation of methanol by Ni3+, it is impossible to transfer electrons through the Ni3+/Ni2+ valence change process.
If the electron transfer leads to the reduction of Ni3+ to Ni2+, it means that there has been a huge fluctuation in the interface energy level, and the electron transfer will seriously deviate from the Marcus electron transfer mechanism.In this study, Yan Shicheng's research group discovered the nucleophilic site of the reaction and the thermodynamic combination criterion of molecular energy levels, which explains the effectiveness of the selectivity of organic electro-oxidation products.
Based on their previously proposed nucleophilic site of organic electro-oxidation reaction and the thermodynamic combination criterion of molecular energy levels, they used density functional theory to calculate the dual local softness value (∆sk) of atoms in the molecule.
The results show that the magnitude of ∆sk can help determine the nucleophilic atomic sites in the molecule. Generally, the more negative the ∆sk value of an atom, the greater the possibility of it becoming a nucleophilic attack site.
The calculation results show that the hydroxyl oxygen in the methanol molecule has a relatively negative ∆sk value, indicating that it is a nucleophilic attack site. At the same time, electron spin resonance spectroscopy confirmed the presence of a large number of unsaturated coordinated Ni sites (oxygen vacancies) in the Ni(OH)2 electrode.
This means that the oxidation of methanol molecules is achieved by establishing an isoenergetic electron transfer channel through the interaction between the hydroxyl oxygen and the unsaturated coordinated Ni3+.In addition, for molecular oxidation reactions, their electrons are injected into the electrode through the energy level of the highest occupied molecular orbital (HOMO, highest occupied molecular orbital).
Therefore, the relationship between the HOMO energy level of the molecule and the Fermi energy level of the electrode can serve as a thermodynamic criterion for whether the reaction can occur.
Theoretical calculations show that the HOMO energy level of the methanol molecule is higher than the Fermi energy level of NiOOH, which means that the chemical potential of the electrons in the HOMO energy level of the methanol molecule is high enough to drive the injection of electrons into the Fermi energy level of NiOOH, enabling the oxidation of the methanol molecule.
On the other hand, the HOMO energy level of the formic acid molecule is much lower than the Fermi energy level of NiOOH, thus it can exist stably as a product.
Electrochemical experiments confirm that, similar to the oxidation of methanol to formic acid, Ni3+ can effectively oxidize a variety of primary alcohols (methanol, ethanol, propanol, butanol, benzyl alcohol) to the corresponding carboxylic acids.For this purpose, the team, considering that the (HOMO, Δsk) combination successfully explained the physical origin of the selectivity in the methanol-formic acid reaction, attempted to investigate the applicability of this combination of parameters in judging the selectivity of various primary alcohol oxidation reactions.
Theoretical calculations show that the hydroxyl oxygen of primary alcohols is a nucleophilic atomic site, and the HOMO energy levels of the examined primary alcohols are all higher than the Fermi energy level of NiOOH, which represents that the electrochemical oxidation reactions of these alcohols are thermodynamically allowed processes.
However, the product selectivity can be divided into two types: Δsk-controlled and HOMO-controlled. The HOMO energy levels of formic acid, acetic acid, propionic acid, and butyric acid are all deeper than the Fermi energy level of NiOOH, belonging to the HOMO-controlled type reactions; while the HOMO energy level of benzoic acid is very close to the Fermi energy level of NiOOH, but its carboxyl oxygen's Δsk value is positive.
This is because the benzene ring is a strong electron-withdrawing group, which thus deactivates the nucleophilic atoms in the carboxyl group, making the Δsk value positive, weakening the nucleophilic effect and making benzoic acid stable as a product, becoming a Δsk-controlled reaction.
It can be seen that this study proves that the (HOMO, Δsk) combination can serve as an effective criterion for the occurrence of organic electro-oxidation and product selectivity.In general, this discovery aims to visualize the oxidation of primary alcohols to carboxylic acids catalyzed by nickel-based catalysts, and to resolve the current debate on the electron transfer mechanism that is receiving much attention.
This finding has a very high correlation with molecular descriptors, providing a clear and understandable prediction of the feasibility of nickel-based primary alcohol electro-oxidation.
At present, the highly selective oxidation of primary alcohols to carboxylic acids has significant application value in the production of bulk chemicals and the fine chemical industry.
Especially under the "dual carbon" strategy background, the development of high product selectivity primary alcohol-carboxylic acid oxidation technology can achieve zero carbon emission chemical preparation and hydrogen production coupling, which has an important application prospect in the field of large-scale energy storage.
Specifically, by screening the alcohol-to-carboxylic acid reaction and developing efficient energy conversion nickel-based catalysts, an efficient and energy-saving two-electrode device with high added value products can be constructed.In the process, anodic electrochemical oxidation of alcohols generates acids, and cathodic reduction of hydrogen ions produces hydrogen gas. Compared to electrochemical water splitting, the liquid product at the anode replaces oxygen, increasing the added value of the product, and the gaseous hydrogen at the cathode is easier to separate, thereby simplifying the energy conversion device.
Looking back on the whole process, the entire research process can be divided into four stages:
Firstly, the phenomenon was discovered.
The team's doctoral student, Yan Yuandong, found the experimental phenomena very interesting during his exploratory research on the electro-oxidation of organic compounds.
In stark contrast to the oxidation of water, which requires Ni with a valence of +(δ > 3, around 1.5V), a series of organic compounds such as urea and alcohols can directly undergo molecular electro-oxidation at the Ni2+/3+ valence potential (around 1.32V).This has provided the research team with an important inspiration: there are potentially significant differences in the reaction mechanisms and patterns between the electro-oxidation of nickel-based organic compounds and water oxidation.
The source of these differences comes from the only two variables—the intrinsic characteristics of the organic molecules and the interrelationship between the molecular energy levels and the energy levels of the nickel catalyst.
Secondly, knowledge reserves.
In order to clarify the reaction process and intrinsic characteristics of the electro-oxidation of nickel-based organic compounds, they have conducted extensive explorations and gradually formed a set of research methods.
For example, in the process of exploring and clarifying the electron transfer mechanism at the electrochemical interface, they accidentally learned about the dinitrophenylhydrazine molecule, which was originally used to detect and quantify trace amounts of Ni2+, with high sensitivity (detection concentration limit nM, time scale ns).In their study of the electron transfer mechanism at the active center, they precisely needed to detect whether Ni2+ was produced. Consequently, dimethylglyoxime became an important indicator molecule for them, helping them visually observe the presence or absence of Ni2+.
Ultimately, combining other evidence, they proposed a direct electron transfer electrocatalytic reaction mechanism for trivalent nickel.
Secondly, when studying the intrinsic characteristics of nickel-based organic electro-oxidation, they urgently needed a theoretical model that could quantify the intrinsic characteristics of organic molecules and the interrelationship between the molecules and the nickel catalyst.
However, after research, there is currently a lack of a comprehensive theoretical model that adequately describes the nucleophilic reaction sites at the atomic scale of organic molecules and the thermodynamics of molecular energy levels.
After further exploration, they proposed a combined criterion of nucleophilic sites and molecular energy level thermodynamics for organic electro-oxidation reactions [1].In it, the magnitude of the dual local softness value (∆sk) of atoms within a molecule can help determine the nucleophilic sites of the atoms in the molecule. As previously stated, the more negative the ∆sk value of an atom, the greater the possibility of it becoming a nucleophilic attack site.
For molecular oxidation reactions, the electrons are injected into the electrode through the highest occupied molecular orbital (HOMO) energy level. Therefore, the relationship between the HOMO energy level of the molecule and the electrode Fermi energy level can serve as a thermodynamic criterion for whether the reaction can occur.
Third, result analysis.
The highly selective oxidation of primary alcohols to carboxylic acids has important application value in the preparation of bulk chemicals and the field of fine chemicals, so they systematically studied it using the method constructed earlier.
In the study, they were pleasantly surprised to find that the (HOMO, ∆sk) combination, as a criterion for the selectivity of Ni3+ catalyzed alcohol-carboxylic acid reactions, can divide the product selectivity into two types: ∆sk dominated and HOMO dominated, which is highly consistent with theoretical analysis and experimental results.Finally, things fall into place.
Rome wasn't built in a day, and every small step forward involves a great deal of effort from researchers. It is both standing on the shoulders of many outstanding electrochemists from the past century and on their own efforts that, with the groundwork laid, the results fortunately come to fruition.
At the same time, Yan Shicheng believes:
Firstly, research requires adaptability.
For example, when analyzing the product, they needed to analyze the formic acid yield in the alkaline solution, but the chromatographic columns they could effectively use were mainly for detecting in a neutral environment.So they titrated the reaction solution to neutrality, then proceeded to test the products, and ultimately obtained the yield of formic acid very smoothly.
Secondly, scientific research requires challenging inertia.
They are well aware that the purpose of scientific research is to discover new knowledge and truth, and this process often requires them to break free from existing thinking patterns and the shackles of concepts to think about problems.
In scientific research, it is necessary to continuously propose hypotheses, conduct experiments, and constantly revise their views. This requires them to maintain an open mindset when facing problems, and to be brave enough to accept the challenges of new things and new ideas.
If you always stay in the original comfort zone and are unwilling to try new methods or viewpoints, then you cannot make real progress.Whether proposing the direct charge transfer mechanism of high-valence active centers in electrocatalytic reactions or proposing the nucleophilic site and molecular energy level thermodynamic combination criteria for the electro-oxidation reactions of organic compounds, they are all trying to break through the inertia of thinking, taking objective experimental results as the foundation and previous theoretical research as a reference, and continuously providing new thinking and cognition to the knowledge base of scientific research.
Ultimately, the relevant paper was published in JACS with the title "Trivalent Nickel-Catalyzing Electroconversion of Alcohols to Carboxylic Acids," with Yan Yuandong as the first author and Yan Shicheng as the corresponding author.
As mentioned earlier, this work takes the oxidation of primary alcohols to carboxylic acids as an example to reveal the rate-determining step, electron transfer mechanism, and product selectivity mechanism of electrocatalytic oxidation reactions of organic compounds.
The types of organic compounds are very rich, and the characteristics of various functional groups are also very different. Exploring the basic laws of electro-oxidation reactions of organic compounds is the basic premise for promoting the application of this technology in the field of large-scale energy storage.
Therefore, they will expand the methodology of this study and continue to elucidate the rate-determining steps, electron transfer mechanisms, and product selectivity mechanisms of various electrocatalytic oxidation reactions of organic compounds.
POST A COMMENT