Recently, a team led by Professor Ning Cunzheng from Tsinghua University and Shenzhen Technology University has conducted research on heat transfer at the nanoscale. By quantitatively measuring the change in interlayer thermal conductivity of two-dimensional materials as a function of interlayer spacing, they systematically analyzed for the first time the different contributions of phonon heat conduction, air heat conduction, and thermal radiation to interlayer heat transfer at a scale of 1-2nm.
The researchers found that under the joint action and trade-off of these three different heat transfer mechanisms, there is an optimal spacing that minimizes the interlayer thermal conductivity of two-dimensional material heterojunctions, which is only two thousandths of the lowest thermal conductivity value of all existing insulation materials, thereby improving the insulation performance of the best existing insulation materials by 500 times.
Ning Cunzheng said: "This indicates that two-dimensional materials are expected to achieve a huge breakthrough in insulation applications. Based on the understanding of heat transfer mechanisms at the nanoscale, we may be able to solve some of the current problems in thermal management."
Advertisement
In addition, the researchers also solved the problem of measurement accuracy in the field of heat transfer at the nanoscale.
For example, in previous studies using Raman spectroscopy to characterize the thermal conductivity of two-dimensional materials, the impact of factors such as doping and strain was often overlooked. This study adopted an improved dual-beam Raman and photoluminescence spectroscopy method to eliminate interfering factors and accurately characterize the thermal conductivity.
Recently, the related paper was published in Advanced Materials with the title "Extreme Thermal Insulation and Tradeoff of Thermal Transport Mechanisms between Graphene and WS2 Monolayers" [1].
Dr. Zhang Ruilin from Tsinghua University and Associate Researcher Gan Lin are the co-first authors, and Professor Ning Cunzheng serves as the corresponding author.The reviewer commented on the study, saying: "This research provides valuable insights into the interlayer heat transfer between graphene and tungsten disulfide, elucidates the basic science of heat transfer mechanisms at the nanoscale, and offers potential impacts for various applications. Similar models can also be applied to other two-dimensional materials, opening up an interesting new method for thermal management."
New Method for Heat Transfer Mechanism Research and Thermal Management
The heat transfer system in this study is a bilayer structure composed of monolayer tungsten disulfide (WS2) and graphene (Gr). The WS2/Gr heterostructure is fabricated by mechanically exfoliating single-layer materials and then stacking them on a silicon substrate through a dry transfer operation.
For an ideal contact WS2/Gr bilayer structure, the interlayer spacing is below 1nm. However, in the actual preparation process, the presence of some bubbles and wrinkles often results in an interlayer spacing of more than 2nm.
To achieve control of the interlayer distance, the research team subjected the samples to high-temperature vacuum annealing, adjusting the annealing time and temperature to achieve samples with different interlayer spacings.
Ultimately, a series of WS2/Gr heterostructure samples with interlayer spacing ranging from complete contact to several nanometers were obtained to systematically study the dependence of interlayer thermal conductivity on spacing. It is noteworthy that the researchers increased the interlayer distance from complete contact to several nanometers to explore the heat transfer mechanism, a level of precision in distance control that is difficult to achieve under normal circumstances.
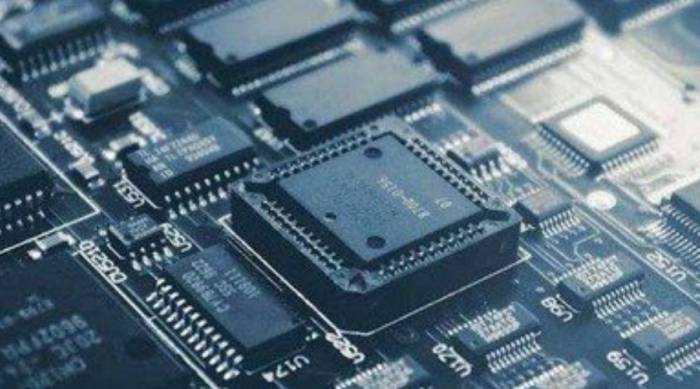
It should be understood that in the WS2/Gr bilayer structure, possible interlayer heat transfer mechanisms include phonon heat conduction, air heat conduction, and near-field thermal radiation.
Researchers found through experimental and theoretical analysis that when the interlayer spacing of WS2/Gr is close to the ideal contact state, interlayer heat transfer is dominated by phonon heat conduction. As the interlayer spacing increases, the phonon thermal conductivity decays rapidly due to the weakening of the coupling degree of phonons between the two materials.On the other hand, the thermal conductivity of air continues to increase with the increase of the spacing until it exceeds the phonon and begins to dominate the interlayer heat transfer. As for the contribution of thermal radiation, it is negligible compared to the phonon and air thermal conduction.
Therefore, under the balance between the two heat transfer mechanisms of phonon thermal conduction and air thermal conduction, there is an optimal layer spacing that makes the interlayer thermal conductivity of WS2/Gr reach the minimum value.
"Being able to discover the subtle rise and fall and balance between different heat transfer mechanisms through the control of interlayer spacing at such a small scale is very meaningful for understanding the basic physical process of heat conduction, and the excellent thermal insulation performance found at this optimal balance point may have important technical applications," said Ning Cunzheng.
In the experimental results, the sample with a layer spacing of 2.11nm has the lowest interlayer thermal conductivity, which is 1.41×10^-5 W/m·K, and this indicator is only two thousandths of the lowest thermal conductivity of the existing reported thermal insulation materials.
"That is to say, our thermal insulation structure is 500 times the thermal insulation performance of the existing best thermal insulation materials, indicating that similar WS2/Gr heterostructures have great potential in thermal insulation," said Ning Cunzheng.
Compared with the macroscopic scale, the challenge of nanoscale heat transfer research lies in the precise control of the nanoscale and the precise measurement of the thermal properties of nanomaterials.
For the study of the flat-plate-to-flat-plate heat transfer model, existing experimental studies mainly control the spacing between two objects through technologies such as nano-column support and piezoelectric actuators, and measure the temperature and heat flow of the objects through integrated electrical heating and temperature sensing devices.
Under this complex experimental setup, the experimental test spacing that can currently be achieved is from 100 nanometers to a minimum of 7nm.
In this study, through methods such as dry stacking and vacuum thermal annealing, the layer spacing of two-dimensional materials can be precisely controlled below 3nm. The flatness and the characteristic of no out-of-plane chemical bonds of two-dimensional materials provide an unprecedented opportunity for the study of interlayer heat transfer.Additionally, due to the materials being highly susceptible to interference from the surrounding environment, their properties and structures can change. Therefore, the accuracy of measuring thermal properties such as thermal conductivity has become a significant challenge.
This study employs non-destructive, pure optical methods such as Raman and photoluminescence spectroscopy to investigate heat transfer, without introducing complex structures such as electrodes, metallic reflective films, and microelectromechanical systems into the material structure, which greatly ensures the stability of the material properties and interlayer structures.
Moreover, this method can exclude the effects of doping, strain, and other factors, thereby accurately extracting the independent impact of thermal effects, ensuring the accuracy of the experimental results.
"Serendipitous" Discovery
In fact, the heat transfer between heterojunctions of two-dimensional materials was not the original intention of the research group's study. While studying the interlayer charge transfer of two-dimensional material heterojunctions, they found that other effects such as strain and heat transfer affected the measurement and understanding of charge transfer. Therefore, it was necessary to disentangle the effects of different factors to accurately measure the heat transfer effect.
However, after repeated analysis and research, the researchers found that the interlayer heat transfer of two-dimensional materials is an important and interesting research direction. This type of double-layer two-dimensional material may be a good thermal insulation structure. By continuously exploring and digging in this direction, they gradually realized and understood the full meaning of the results, leading to today's achievements.
"I think this continuous exploration and adjustment of the unknown, the balance between perseverance and appropriate flexibility, these unexpected and serendipitous discoveries, are the joys of scientific research," said Ning Cunzheng.
So, how can this small-scale thermal insulation structure be introduced into the thermal management of electronic components and precision instruments?Zhang Ruilin explained, "The WS2 side of the WS2/Gr heterojunction can be oriented towards the thermally sensitive unit to be protected, while the Gr side faces the direction where a heat source may exist."
When an external heat source flows in, the heat will dissipate rapidly within the graphene plane (due to the high in-plane thermal conductivity of graphene), and the interlayer direction perpendicular to Gr and WS2 will block the heat flow, thereby protecting the thermally sensitive unit.
The team hopes that similar structures can be used for thermal management in chips or integrated circuits, that is, to dissipate heat from high-temperature heat sources as quickly as possible, while isolating the heat source from key components to prevent overheating.
In addition, this thermal insulation structure can be applied to the surface of handheld or wearable electronic devices to isolate the human skin.
That is to say, when the device is locally overheated, effective heat dissipation is first achieved within the Gr plane, and the heat will be effectively blocked in the interlayer direction from Gr to WS2, thus reducing the damage of electronic device heat to human skin.
It is reported that the size of the two-dimensional material heterostructures prepared by the current research group is in the order of tens of micrometers. In the future, for thermal insulation applications, larger-sized two-dimensional material heterostructures are needed.
Next, on the one hand, researchers plan to study how to achieve large-sized two-dimensional material heterojunctions with clean interfaces and controllable interlayer spacing; on the other hand, they will also explore their application in thermal management of small devices.
POST A COMMENT