Recently, Dr. Gao Hongyan from the University of Massachusetts and his team have constructed a mesh bioelectronic system.
It is a sensor embedded with multifunctional graphene nanoelectronics, capable of tracking the excitation-contraction process of cardiac micro-tissues due to the integration of single-layer graphene transistors.
In this device, graphene acts as a transistor, using graphene's field effect and piezoresistive effect to simultaneously detect the action potential and mechanical signals of cardiac micro-tissues.
Through this, the research team not only integrates multifunctional sensing into the same device but also avoids the challenges of signal synchronization, device addressing, and integration of heterogeneity that exist in traditional strategies.
The flexible mesh network within can provide tissue-level softness and cell-level characteristic dimensions, thereby achieving close contact with cardiac tissue.This allows the device to simultaneously monitor the electrical and mechanical signals within the cardiac micro-tissue, which are the two most important reference signals for the human heart to function properly.
Advertisement
Through the complex excitation-contraction process, these two types of signals can be coupled together. Therefore, the action potential signals and mechanical contraction signals detected at the same location by this device can reflect the state of the cardiac micro-tissue during its development.
It is understood that mature cardiac tissue can exhibit larger action potential amplitudes and mechanical vibration amplitudes, and these indicators can all be reflected by the changes in the electrical conductivity of graphene.
In addition, the research team also uses drug treatment methods to suppress the signals, so the process of signal changes in the device, such as changes in amplitude and frequency, can intuitively reflect the impact of the related drugs on the cardiac micro-tissue.
In summary, this sensing platform allows the entire tissue to stably track the complex excitation-contraction coupling and can span the entire development process of the tissue.At the same time, it can comprehensively evaluate the maturity of cardiac tissue, distinguish the effects of drugs, and perform disease modeling. Compared with single-modality sensor technology, the perception platform in this study can create a rich dataset.
Targeting the function, development, and pathophysiological state of cardiac tissue, it is expected to achieve more accurate quantitative results, thereby providing an important tool for improving cardiac tissue engineering.
It is understood that since the device in this study can be encapsulated within the cardiac micro-tissue, environmental factors that do not affect the cardiac micro-tissue, such as temperature and oxygen content, will not affect the output signal.
At the same time, this study overcomes the limitations of optical imaging sensing and single-modality electronic sensing. By combining bioelectric sensing and biomechanical sensing, it provides a comprehensive assessment of the dynamics of micro-tissue development.
This allows the results of this study to be seamlessly integrated into micro-tissues, achieving minimally invasive and stable tracking of the tissue development process, and thus providing a promising tool for tissue engineering research.Subsequently, if the experimental conditions are further optimized, the current device may also be used for drug screening of three-dimensional cardiac tissues.
For cardiac micro-tissues, it can also provide a micro-environment more similar to the human heart, which is very promising for the monitoring of living cardiac tissues, thereby improving the efficiency of drug screening.
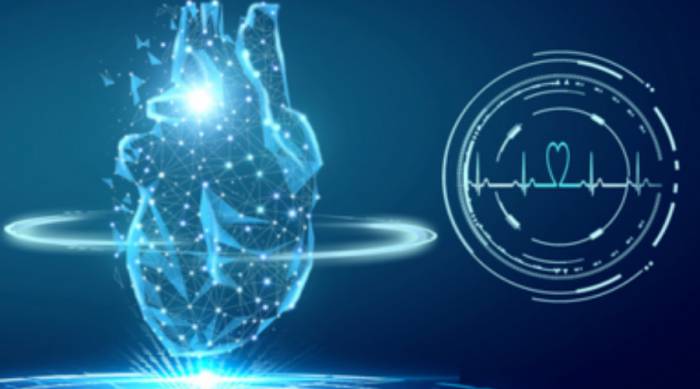
It is also reported that previously, mesh electronics and graphene transistor electronics have been widely used for monitoring living neural signals. The mesh electronics constructed this time provide a tool for intuitively analyzing the side effects of drugs on the heart.
Moreover, due to graphene's high monitoring sensitivity and good chemical stability, it makes it a more suitable material than silicon nanowires.
Overall, the use of graphene can bring the following advantages:Firstly, graphene is a two-dimensional material, which can be uniformly obtained by chemical vapor deposition, providing a basis for large-scale integration and device uniformity.
Secondly, graphene is a material that has both field effect and piezoresistive effect, and it can theoretically monitor changes in voltage and strain at the same time, thereby integrating two functions into the same device.
Thirdly, graphene is also a carbon material with excellent chemical stability, which can achieve long-term physiological monitoring.
At the same time, other two-dimensional semiconductor materials can also be used to replace graphene, provided that this material has both piezoresistive and field effects, as well as physiological environment stability and the ability to synthesize on a large scale.The Road to Monitoring Cardiac Microtissues: A Long and Arduous Journey
As previously mentioned, this achievement can be used for the detection of cardiac signals. Heart diseases are one of the main causes of illness and death in humans. The lack of animal models makes in vitro cardiac tissues an important alternative choice.
Among them, cardiac tissues constructed from human stem cell-derived cardiomyocytes are the most commonly used in vitro models. They can retain patient information, thus being used for the study of genetic diseases and personalized drug screening.
Compared with two-dimensional tissue culture, three-dimensional cardiac microtissues are the preferred tissue models because they can better reproduce cell phenotypes, microenvironments, and cell interactions, presenting experimental effects close to those of living organs.
For monitoring the development status of cardiac microtissues, electrical signals and mechanical signals are two of the most intuitive parameters that can be used for the evaluation of drug effects and diseases.For the electrical and mechanical activities of cardiac micro-tissues, they are essentially interconnected through excitation-contraction coupling. Therefore, simultaneously measuring the relevant dynamics is extremely important for the study of cardiac micro-tissues.
For example, in patients with chronic myocardial infarction, the dysfunction of their cardiac cells is correlated with impaired excitation-contraction coupling. At the same time, many arrhythmias are also caused by weakened excitation-contraction coupling.
Therefore, spatial mapping conducted solely by tracking electrical responses or mechanical responses has certain limitations in identifying impaired excitation-contraction coupling links.
In research related to development, the maturity or aging of tissues is usually more suitable to be characterized by the dynamics of excitation-contraction coupling, rather than just by information from electrical or mechanical activities.
Previously, people used optical means to track electrical activities and mechanical movements, and then applied them to three-dimensional micro-tissues.However, due to the opacity of the tissue, only superficial physiological activities can be obtained.
Using electronic sensors integrated on planar or flexible substrates also faces the same issue.
Despite the development of flexible mesh electronics, which provides opportunities for embedding addressable sensors in deep tissues, especially the flexible ribbon features that make the mechanical properties of mesh devices close to the tissue, thereby reducing invasiveness and extending the stability of the interface.
However, existing platforms can only detect a single mode of electrical or mechanical activity, which is indeed sufficient to detect tissues such as nerves, but not enough to characterize the mechanical and electrical responses related to the cardiac system.
It is known that due to the presence of cardiotoxic side effects, about one-third of drugs cannot achieve clinical application.Previously, the team has confirmed that three-dimensional silicon nanowire transistors constructed through micro-nano processing technology can be made into dual-functional electronic devices capable of measuring planar monolayer myocardial cells simultaneously with the help of field effect and piezoresistive effect [1].
However, the system is not applicable in cardiac micro-tissues, mainly because it is difficult to assemble three-dimensional silicon nanowires on an independent substrate in a bottom-up manner.
Combining cells with electronic devices ingeniously
The idea of conducting electromechanical monitoring of cardiac micro-tissues originated from Gao Hongyan's previous research on silicon nanowire transistors.During the previous project, he found that single-layer cells often detached from the base and spontaneously curled up, then folded into three-dimensional micro-tissues.
For the monitoring of single-layer cell signals, this is undoubtedly an unfavorable factor, as the separation of the device from the cells will directly lead to the interruption of signals.
After communicating with his mentor, Gao Hongyan formed the following idea: to construct an electronic device that embeds directly into the heart tissue along with the curling and folding of cells to monitor mechanical and electrical signals.
Following this idea, as well as the experimental feasibility provided by the previous silicon nanowire project, Gao Hongyan began to design the device.
As a more traditional transistor, graphene has been widely used to monitor cellular action potentials. However, the monitoring of cellular mechanical activity by graphene is still in a research vacuum.The reason lies in the fact that graphene is a single-layer structure of carbon atoms with a diameter of only about 0.3nm. Therefore, special attention needs to be paid to the damage of graphene during the transfer process and its contact with metal electrodes during device processing.
Additionally, since the graphene transistor needs to be constructed on another polymer surface with a thickness of only 400nm, the processing process is also more delicate.
At the same time, how to achieve the integration of flexible mesh devices with cardiac micro-structures is also a challenge faced by this research. Moreover, the ultra-thin mesh devices will bring a lot of uncertainty to the operation process, resulting in a very low yield of devices in the early stage.
Later, by optimizing the cell culture process and optimizing the integration method, and then fusing the fixed mesh devices on the substrate with myocardial cells, the experimental efficiency was finally greatly improved.
Finally, the relevant paper was published in Nature Communications with the title "Graphene-integrated mesh electronics with converged multifunctionality for tracking multimodal excitation-contraction dynamics in cardiac microtissues" [1].Gao Hongyan is the first author, and Professor Yao Jun from the University of Massachusetts serves as the corresponding author.
In general, this study demonstrates that the interface between reticulated electronics and three-dimensional biological tissues can provide a wealth of biological information.
Additionally, the study employed in vitro experiments, so can the conclusions drawn from in vitro experiments truly reflect the behavior of tissues in vivo?
In response to this, the team stated that the experiments used micro-tissues constructed from cardiomyocytes differentiated from human embryonic stem cells, which can reflect the development process of myocardial tissue to a certain extent.
However, due to the complex multi-level structure and function of the in vivo heart, there is still a certain gap in truly reflecting in vivo behavior.Therefore, the team will further explore the application of this achievement in analyzing physiological signals of three-dimensional biological tissues in the future.
POST A COMMENT